Glucose is a paradox, best personified as a soccer hooligan. He arrives late for the important match with his mates after the ground is full and is not able to get through the turnstiles. Had he been more punctual, his exuberant energy would have been expended to drive his team over the line. Now this energy is released in other areas, creating havoc in the form of black eyes in pubs, overturned tables in cafes and broken windows. This analogy will become obvious as this article unfolds.
Diabetes affects more than 422 million people worldwide (WHO 2014). It is the leprosy of the 21st century. It causes microvascular disease, resulting in potential loss of limb, vision and renal function. On a different level, it accelerates macrovascular disease, a condition not exclusive to diabetics, but certainly augmented by hyperinsulinaemia due to insulin resistance, resulting in syndrome X. In gestational diabetes it has its own unique set of circumstances, with the fetus the focus of management. A large proportion of the health budget is spent on the prevention, diagnosis and management of this disease. Likewise, a significant portion of our clinical thinking time goes into managing patients with diabetes. We do understand the diagnosis, management and effects of this condition, but do we really understand why the seemingly harmless glucose molecule potentially causes so much damage?
Sugar connotes happiness, good times and contentment. How can such a nice substance cause such devastation? Glucose is the only brain fuel and is essential to the normal functioning of every living cell. However, we all know that we have to avoid excess sugar for our own good. As doctors we tell our diabetic patients to keep their blood sugar levels down, so they don’t get the nasty complications of the disease, but do we really stop to consider what it is about glucose that makes it potentially so harmful? To refresh our memories, we need to go back to biochemistry in the first year of our medical studies.
The biochemistry
Glucose is intrinsic to cellular respiration. This complex series of enzymatic equations can be summarised thus: C6H12O6 + 6O2 -> 6CO2 + 6H2O + energy (captured in the form of 38 adenosine triphosphate [ATP] molecules). This is the exact reverse of photosynthesis.
The glucose molecule is a complex molecule largely due to its stereochemistry, but essentially exists in two forms. There is the inert ring structure and the chemically active linear structure (Figure 1). It is important to notice how similar glucose is to sorbitol, another carbohydrate.
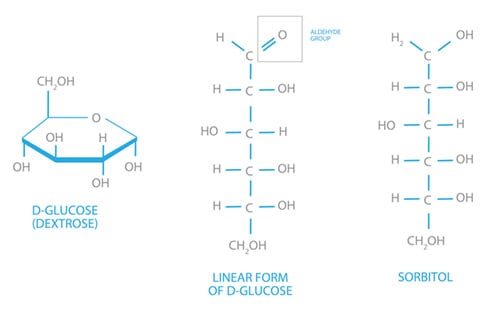
Figure 1. The two common isomers of glucose and sorbitol.
Cellular respiration is an exothermic reaction, of which the important end product is energy, harnessed in ATP molecules. One glucose molecule produces 38 ATPs and these are the ‘alkaline energisers’ for every cell in the human body. This is a complex process and occurs via glycolysis, the Krebs cycle and oxidative phosphorylation.
In the cytoplasm of each cell, each glucose molecule (six carbons) is split into two pyruvate molecules (three carbons) via a series of enzymatic equations we call glycolysis. In this process, it produces a net two ATP molecules. Each pyruvate molecule acts as a substrate for the Krebs (citric acid) cycle. It enters the outer mitochondria and is converted to acetyl coenzyme A (acetyl CoA), which triggers the start of the citric acid cycle. In a cyclic series of complex energy-releasing reactions, citric acid is converted back to itself. The energy is captured in 2GTPs (similar to ATP), 10 NADHs and 2 FADHs. The latter two molecules are important cofactors in the electron transport chain, also known as oxidative phosphorylation, which occurs within the inner matrix of the mitochondria. They act as proton (hydrogen ion) donors and release electrons in this serial oxidative cascade. In this process, a hydrogen ion gradient is created across the inner mitochondrial membrane. To restore the osmotic balance, the hydrogen ion flux drives the ATPase enzyme, hence phosphorylating ADP to form the high energy ATP. Electrostatic neutrality is achieved by oxygen accepting electrons to form water. This is the only reason we breathe oxygen! Without it cellular respiration grinds to a halt. All in all, one glucose molecule produces a total of 38 ATPs, equivalent to 1272 kilojoules (304 kilocalories) of energy per mole of glucose metabolised.
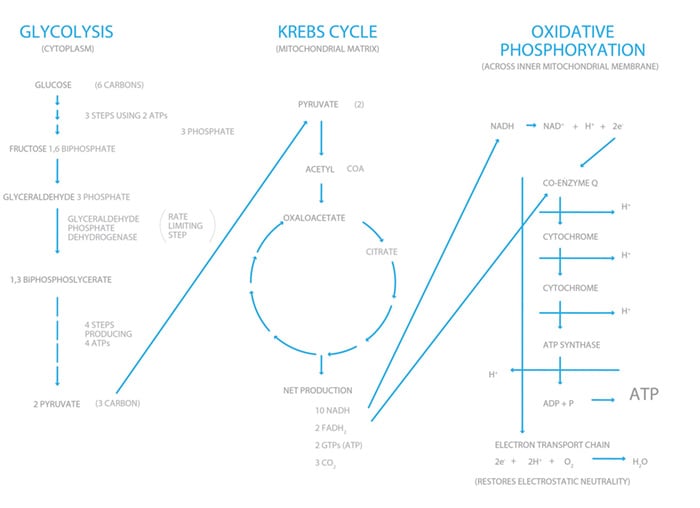
Figure 2. Very simplified version of cellular respiration.
So far so good, all the spectators have been accommodated and they can cheer on their teams. Their energy is directed for a positive good, but what happens when the turnstiles are shut?
As alluded to earlier, diabetics have an increased risk of macrovascular disease. As the HbA1c rises from 5.5 to 9.5, the risk of this complication is doubled. Peculiar to diabetes, however, a similar rise in HbA1c increases the risk of microvascular disease ten-fold. Why is this so? We know that when diabetics are hyperglycaemic, all cells in the body are bathed in glucose and most cells can excrete the glucose and restore homeostasis, but significantly, endothelial cells lack the capacity to do this.1 The cells become damaged and this affects blood flow through the glomeruli in the kidney, retinal blood vessels and vasa nervorum. In turn, this results in chronic kidney disease, retinopathy and neuropathy.
Why is it so?
The mechanisms by which hyperglycaemia results in cell damage have been gradually worked out since the 1970s, but there is still much to learn. There are four abnormal pathways so far identified, all involving side branches of glycolysis due to the flooding of the ‘football stadium’ of oxidative phosphorylation with glucose metabolites, which results in the overproduction of superoxide. This negatively feeds back, inhibiting the rate-limiting enzyme of glycolysis GADPH, which in our analogy would be the turnstile. The hooligans (excess glucose) now turn their abundant energy to other less useful and damaging directions.
The four abnormal pathways to cell damage:
- Increased polyol pathway activity.2
There is an important enzyme in all cells called aldose reductase. This reduces toxic aldehydes (reactive oxygen species) to inactive alcohols (=O to -OH). Glucose is essentially an aldehyde with a terminal double bond O. At normoglycaemia, it only has a 1 per cent affinity for glucose, whereas in hyperglycaemia, this affinity rises to 33 per cent. By this pathway, glucose is converted to sorbitol and then to fructose. In doing so, it redeploys NADPH, an enzyme essential to activate glutathione, a very important anti-oxidant, which keeps the cell in a stable, reduced state. The end result of glucose being diverted into this pathway is increased cellular oxidative stress. - The production of advanced glycation end products (AGEs)3
These form endogenously throughout life from the embryonic stage, but can be taken exogenously in one’s diet. Their formation is accelerated in hyperglycaemia. They are a heterogeneous group of molecules, formed by glucose binding covalently to and between proteins. As a harmless example, HbA1c is produced by this mechanism. These can alter the structure and function of affected tissues, for example, renal and retinal tissue.
Receptors for AGEs (known as RAGEs) are found throughout most tissues, particularly mast cells, and these stimulate production of pro-inflammatory cytokines, pro-coagulants and vasoconstriction, resulting in tissue and blood vessel damage. - Activation of protein kinase C (PKC)4
PKCs are a family of enzymes involved in controlling the function of other proteins by phosphorylation. There are 12 isoforms and their production is stimulated by intracellular levels of diacylglycerol (DAG). These enzymes build up by inhibition of the enzyme GAPDH, the controlling enzyme in glycolysis, the soccer turnstile so to speak.Through the increased production of PKCs, more phosphorylation occurs, activating enzymes not normally activated in more quiescent times, for example: PDGF (platelet-derived growth factor), which cause cellular apoptosis; increased VEGF (vascular endothelial growth factor); reduction in production of nitric oxide which causes vasoconstriction; as well as multiple other adverse effects. - Increased flux into the hexosamine pathway5
This pathway is increased by a factor of 2.4 in hyperglycaemia and usually uses up only three to five per cent of total glucose. Fructose-6-phosphate, the second step in glycolysis, instead of converting to fructose 1,6-biphosphate is converted to glucosamine-6-phosphate, which binds to the base uridine to form uridine diphosphate N-acetylglucosamine (UDP-GlcNAc). This gets incorporated into messenger RNA (mRNA), producing the cytokine proteins TGF (alpha and beta) and plasminogen activator 1. This is one of the pathways thought to damage pancreatic beta cells, hence increasing insulin resistance.
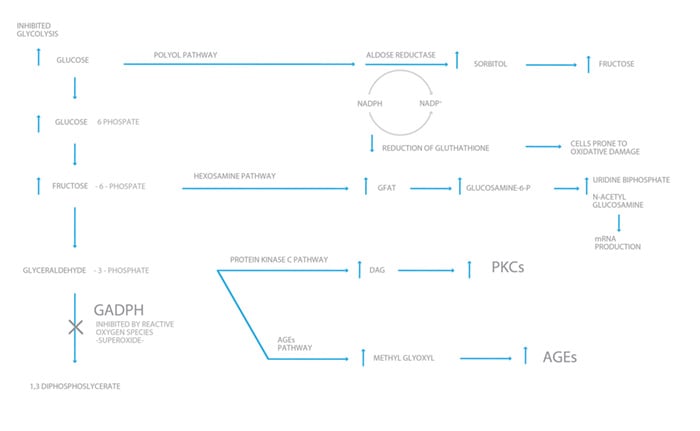
Figure 3. The pathways to cellular destruction.
These four pathways are all activated by hyperglycaemia, which overloads the electron transport chain (the football stadium), resulting in an overproduction of superoxide and reactive oxygen species. These negatively feedback on the rate-limiting enzyme in glycolysis GAPDH (the turnstile), causing an increase in all products upstream and the fluxing of glucose (the redeployed soccer hooligans) into the four previously described pathways. The resulting oxidative stress causes tissue damage by all the mechanisms listed above. Without hyperglycaemia, none of this damage would occur. With freer turnstiles, the broken windows, overturned tables and black eyes would have been prevented!
The epidemiology
This discussion has been all at a cellular level, but apart from our own clinical observation, how do we know that all of these complications from this nice but nasty glucose molecule occur at a population level? In the late 1970s, two important landmark studies, which due to prohibitive cost will never be repeated, were commenced. These were the Diabetes Control and Complications Trial (DCCT)6 and the United Kingdom Prospective Diabetes Study (UKPDS).7 The DCCT dealt with the early aggressive management of type 1 diabetes and the UKPDS addressed type 2 diabetes. Both proved that, with almost 20 years of observation, intensive glucose control resulted in fewer microvascular complications.
In the UKPDS8 it was found that, for every point reduction in HbA1c (for example, from nine to eight), there was a 35 per cent reduction in complications. It was also established at the ten-year review that the reduction in complications was dependent upon early glycaemic control. Those with early poor control never achieved the same reductions as those with meticulous control from diagnosis, even if it slackened off after the trial finished. This is known as the legacy effect, due to glycaemic memory, and the mechanism is most likely epigenetic. Similarly, this is where the long-term effects of gestational diabetes arise.
Epigenetics
So what is epigenetics? It’s certainly a 21st century buzzword. For a detailed explanation see O&G Magazine Vol 18 No 2, page 22. In a nutshell, it means above genetics, therefore outside the normal paradigm of what we understand genetics to be. Epigenetics is a stably heritable phenotype resulting from changes in a chromosome without alterations in the DNA sequence. The human haploid genome contains three billion base pairs, but genes only account for 15 per cent of these. In each cell there is 1.8 metres of DNA. To be able to squeeze this length of DNA into every cell’s nucleus, it has to be spooled around proteins called histones. The supercoiling involved is able to reduce the length of the DNA to 0.09nm. The way it is spooled affects gene expression for that particular cell and gives it this characteristic function. The spooling can be affected by exogenous factors such as hyperglycaemia. Methylation at certain base pairs can turn genes off and this is the most understood mechanism. How hyperglycaemia does this is not fully understood.
The Barker hypothesis extended
In the same way that Professor David Barker hypothesised in the 1980s that poor fetal nourishment resulted in higher cardiovascular morbidity later in life, there is accumulating evidence that gestational diabetes leads to epigenetic changes, both in the mother and her infant. This can lead to a predisposition to obesity, type 2 diabetes (in mother and baby), as well as cardiovascular morbidity. So like a soccer hooligan, hyperglycaemia causes chaos at a number of levels. It induces hyperinsulinaemia, it causes tissue damage in its own right and induces potentially permanent epigenetic changes. So the moral of this tale is to keep those turnstiles open by maintaining normoglycaemia.
Further Reading
- Holman R, et al. 10 year follow up of intensive glucose control in type 2 diabetes. NEJM 359:15 Oct 2008.
- Hall Stephen S. Small and thin. The New Yorker. Nov 19 2007
- Nyengaard JR, Ido Y, et al. Interactions between hyperglycaemia and hypoxia: implications for diabetic retinopathy. Diabetes. Nov 2004.
- Giacco F, Browlee M, et al. Oxidative stress and diabetic complications. Circulation Research 2010;107:1058-1070.
- Jayaraman S. Epigenetic mechanisms of metabolic memory in diabetes. Circulation Research 2012;110:1039-1041.
- Moen G, Sommer C, et al. Epigenetic modifications and gestational diabetes: A systematic review of published literature. Manuscript for European Journal of Endocrinology Feb 2017. EJE -16-1017.
- El-Osta A, Brasacchio D, et al. Transient high glucose causes persistent epigenetic changes and altered gene expression during subsequent normoglycaemia. The Journal of Experimental Medicine.
References
- Melmed S, Polonsky K, et al. Williams Textbook of Endocrinology. 12th Edition 2011, p1463.
- Browlee M. The pathobiology of diabetic complications: A unifying mechanism. Diabetes June 2009,Vol 54, no 6 P 1616.
- Melpomeni P, Urinarri, et al. Glucose, advanced glycation end products and diabetes complications: What’s new and what works. Clinical Diabetes 2003 Oct:21(4):187.
- Geraldes P, King GL. Activation of protein kinase C isoforms and its impact on diabetic complications. Circulation Research.2010;106:1319-1331.
- Buse M. Hexosamines, insulin resistance and the complications of diabetes: current status. Am J Physiological Endocrinology and Metabolism. 2006 Jan:290(1): E1-E8.
- Diabetes Control and Complications Trial Research Group: The effect of intensive treatment of diabetes on the development and progression of long-term complications in insulin-dependent diabetes mellitus. N Engl J Med. 1993:329:977-986.
- UK Prospective Diabetes Study Group: Intensive blood glucose control with sulphonylureas or insulin compared with conventional treatment and risk of complications in patients with type 2 diabetes mellitus (UKPDS 33) Lancet. 1998;352:837-853
- UK Prospective Diabetes Study Group: Intensive blood glucose control with sulphonylureas or insulin compared with conventional treatment and risk of complications in patients with type 2 diabetes mellitus (UKPDS 33) Lancet. 1998;352:837-853
Leave a Reply