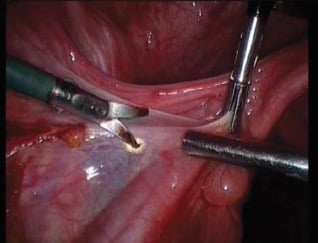
Figure 1. Monopolar scissors – tissue effect vaporisation (non-contact mode, cut waveform).
Today’s surgeons are spoilt for choice when it comes to laparoscopic energy sources, a market where there has been significant change over the last decade. Furthermore, new instruments often arrive accompanied by much fanfare and hype. Unfortunately, many of the laboratory and clinical data on new energy sources are from studies undertaken, or sponsored by, the manufacturer; data from randomised trials are often unavailable.
Irrespective, it remains the responsibility of the surgeon to acquire knowledge on the range of tissue effects available with various laparoscopic energy sources, how these devices impart their tissue effects, and the associated benefits and risks for each device. Hence, it is not an easy task for surgeons to make decisions about the energy sources they choose to use for operative laparoscopy. To this end, the American College of Surgeons has introduced a compulsory laparoscopic energy source module for trainees – Australasia will probably follow suit in the not-too-distant future.
This article provides a summary of the main laparoscopic energy sources used in Australasia; how they work, what advantage(s) they provide over other energy sources and how they can be used safely. An attempt is also made to crack the old chestnut of which laparoscopic energy source is best.
Table 1. The main classes of laparoscopic energy sources and their tissue effects.1
Energy source | Tissue effects |
---|---|
Monopolar electrosurgery | Vaporisation, fulguration, desiccation, coaptation* |
Conventional bipolar electrosurgery |
Desiccation, coaptation |
Advanced bipolar electrosurgery** |
Desiccation, coaptation, blade tissue transection |
Ultrasonic technology | Desiccation, coaptation, mechanical tissue transection |
*Vessel sealing achieved with coagulation and compression.**Tissue impedance monitoring optimises activation time.
Tissue effect | Surgical effect | Current waveform | Contact with tissue | Characteristics |
---|---|---|---|---|
Vaporisation | Cutting | Continuous (cut) | No contact | Low-voltage sparks, moderate smoke |
Fulguration | Haemostasis of small vessels (<1mm) | Interrupted (coag) | No contact | High-voltage sparks, significant smoke and charring |
Desiccation | Haemostasis of small vessels (<1 mm) | Continuous (cut) or interrupted (coag) | Contact | Similar action to bipolar electrosurgery, pronounced lateral thermal spread |
Coaptation | Sealing of small to medium vessels (<2mm) | Continuous (cut) or interrupted (coag) | Contact and compression of vessel wall | Similar action to bipolar electrosurgery, pronounced lateral thermal spread |
Table 2. Monopolar electrosurgery tissue effects.2
Monopolar electrosurgery
All electrosurgery is ‘bipolar’ inasmuch as the electrical current flows from one pole or electrode to another. In monopolar electrosurgery, the active electrode is one pole and the patient return electrode is the other. The main difference between monopolar electrosurgery and the other energy modalities is that electrical current flows through the patient. This difference avails the greatest range of tissue effects to monopolar electrosurgery.3 4
The tissue effects available with monopolar electrosurgery include vaporisation (tissue destruction and cutting), fulguration (tissue destruction and small vessel haemostasis), desiccation (cell wall rupture and cytoplasm boiling) and coaptation (vessel sealing owing to denaturation and renaturation of proteins) (see Figure 1; Table 1).5 These tissue effects are primarily achieved using the ‘cut’ or ‘coag’ electrosurgical unit (ESU) settings while contacting or not contacting the target tissue (see Table 2).6 Varying other parameters under the surgeon’s control, such as power setting, duration of activation and electrode configuration, can further modify the desired tissue effect.7 8 9The scissors configuration in monopolar surgery allows the greatest tissue dissection capability, adding tissue plane dissection and cold cutting to the repertoire.10
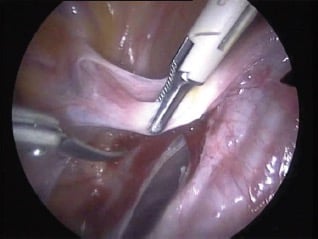
Figure 2. Bipolar forceps – tissue effect desiccation.
All energy sources generate tissue temperatures well above 45°C, the temperature at which irreversible cell damage occurs. Tissue temperatures with monopolar electrosurgery are ~100°C, 100–200°C and >200°C for desiccation (and coaptation), vaporisation and fulguration, respectively. Other laparoscopic energy sources are limited to tissue effects of desiccation and coaptation, and they also generate tissue temperatures of ~100°C.11 12
The major disadvantage of monopolar electrosurgery is the rare but unavoidable risk of stray current injury (SCI). These injuries are often not noticed during surgery as they usually occur outside of the surgeon’s field of vision. They are not owing to surgeon error or lack of skill. Instead, the physics (that is 100 per cent reproducible) is to blame. Used in contact mode, there is the risk of lateral thermal spread injury to adjacent structures with monopolar electrosurgery, just as for all energy sources that yield tissue effects of desiccation and coaptation. Smoke production during monopolar electrosurgery may be problematic, especially during fulguration.13
So, monopolar electrosurgery is a relatively inexpensive, readily available and versatile energy source that yields the best range of tissue effects. Despite the small risk of SCI, this modality remains in common use in conventional laparoscopy, as well as in robot-assisted and single-port laparoscopy.
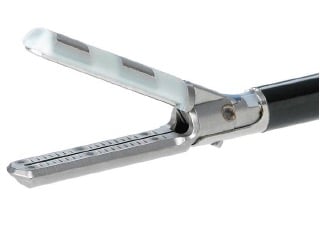
Figure 3. LigaSure (blunt tip) advanced bipolar
device. A cutting blade is incorporated into the
instrument tip.
Conventional bipolar electrosurgery
In bipolar electrosurgery (including advanced bipolar modalities), the active and return electrodes are the two jaws of the energy source. In 1974 Rioux and Cloutier, in North America, and Frangenheim, in Germany, introduced bipolar electrosurgery as a means of eliminating the risk of SCI that had been observed with monopolar electrosurgery, while at the same time a means of sealing larger vessels was born.14 In bipolar electrosurgery, electrical current passes through the tissue held between the jaws of the instrument, not through the patient, and results in tissue desiccation and vessel coaptation in an analogous fashion to monopolar electrosurgery performed in contact mode. Alternating current is standard output for ESUs and it is this physical property that results in efficient sealing of vessels with bipolar electrosurgery, via change of direction of current flow through the tissue compressed between the instrument jaws, as orientation of the active and return electrodes rapidly alternates (see Figure 2).15
A major advantage of conventional bipolar over monopolar electrosurgery is the ability to seal (coapt) vessels up to ~5mm in diameter (monopolar contact mode and fulguration mode would generally be reserved for vessels 1–2mm and <1mm in diameter, respectively). The dissection capability of the bipolar forceps is good, especially in the Maryland configuration. Bipolar electrosurgery is generally available and relatively inexpensive. Disadvantages of bipolar electrosurgery include: lateral thermal spread that will continue until device activation is ceased; no audio signal from the ESU to inform the surgeon when desiccation or coaptation is complete, which increases the risk of injury from lateral thermal spread as well as tissue charring and tissue adherence to the instrument jaws; and the need for another instrument, such as a laparoscopic scissor, for tissue cutting.16

Figure 4. EnSeal advanced bipolar device. Note the retracted copper-colored transection blade at
the jaw hinge.
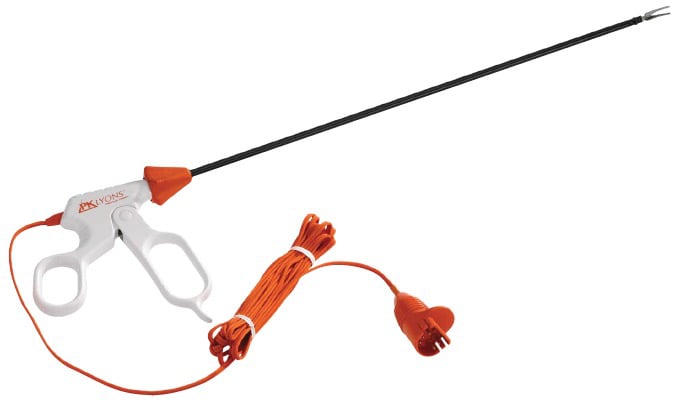
Figure 5. PKS Lyons Dissecting Forceps advanced bipolar device. This is non-bladed, although other
instruments in this range offer a cutting mechanism.
Advanced bipolar electrosurgery
In addition to the features of conventional bipolar electrosurgery, advanced bipolar energy sources are revolutionary in several ways. Firstly, a propriety ESU using a computer-controlled tissue feedback system controls each device, the main players in the Australasian market being LigaSure (see Figure 3; Covidien), EnSeal (see Figure 4; Ethicon) and PlasmaKinetic System (PKS), that is, Lyons Dissecting Forceps (see Figure 5; Gyrus ACMI). The tissue impedance is monitored with continuous adjustment of the generated voltage and current to maintain the lowest possible power setting to achieve the desired tissue effect, at which time an audio signal alerts the surgeon that the endpoint has been reached. In this way, the risk of lateral thermal spread as well as charring of the tissue and adherence of tissue to the device jaws (all of which are more likely with prolonged device activation) is minimised.17
Secondly, these energy sources were the first to be approved by the US Food and Drug Administration (FDA) to seal vessels up to 7mm in diameter owing to technological advances such as: tissue impedance monitoring up to 4000 times per second (LigaSure); temperature-sensitive material in the device jaws that optimises tissue temperatures at ~100 °C (EnSeal); delivery of pulsed energy with continuous feedback control to prevent tissue overheating (PK System); and jaw design that optimises mechanical compression to the vascular pedicle (LigaSure, EnSeal).18 19 Although the ability of these newer devices to seal vessels up to 7mm in diameter is unquestioned, the expected minimisation of lateral thermal spread injuries owing to these technologies has yet to be proven in clinical trials.
The tissue effects available with advanced bipolar electrosurgery are owing to the conversion of electrical energy into thermal energy and include tissue desiccation and coaptation (vessel sealing), just as for conventional bipolar electrosurgery and contact monopolar electrosurgery.20 21 Some devices incorporate a cutting blade into the device jaws (LigaSure, EnSeal) that decreases the need for a laparoscopic scissor. The bulky jaws of such devices, however, are generally inferior dissectors compared to Maryland forceps. Indeed, the PKS Lyons forceps has the advantage of advanced bipolar electrosurgery with good dissection capability, but does require an additional laparoscopic scissor for tissue cutting. Hence, the decision to use a particular bipolar device may come down to dissection capability versus instrument traffic. Although advanced bipolar energy sources are relatively expensive, they are generally available in most hospitals.22
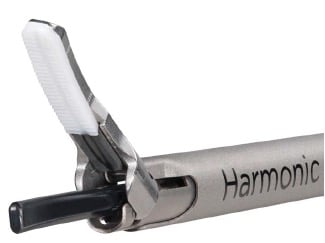
Figure 6. Harmonic ACE+ ultrasonic energy
source.
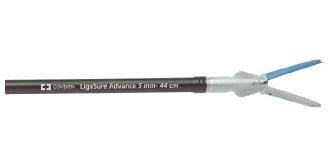
Figure 7. LigaSure Advance hybrid monopolar
and bipolar energy source. The monopolar
active electrode is at the end of the instrument’s
blue jaw.
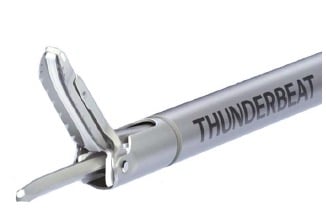
Figure 8.Thunderbeat hybrid ultrasonic and
advanced bipolar energy source.
Ultrasonic devices
The first ultrasonic energy source was described in 1993 by Amaral, called the ‘laparoscopic scalpel’; it had the dual functionality of tissue cutting and vessel sealing.23 Ultrasonic energy sources convert electrical energy into vibrations in the handpiece of the device at frequencies more than 20 000 cycles per second, that is, above the audible range. These vibrations oscillate the non-articulating jaw of the instrument. Tissue is compressed between an articulating jaw and the nonarticulating jaw to impart the tissue effects derived from combination of thermal and mechanical energy: desiccation and vessel sealing is achieved at lower frequencies; and tissue cutting occurs at higher frequencies. The tissue effects arise owing to the conversion of electrical energy into thermal energy for tissue desiccation and vessel sealing, and also mechanical energy for the tissue cutting. As for the other energy sources, the generated tissue temperature is ~100°C to achieve dessication and coaptation; hence, lateral thermal spread injury is possible with ultrasonic energy sources.24
The laparoscopic ultrasonic devices in the Australasian marketplace include the Harmonic ACE+ (see Figure 6; Ethicon) and Sonocision (Covidien), which are both rated by the FDA to seal vessels 5mm in diameter. The Harmonic ACE+ has ‘Adaptive Tissue Technology’ that provides an audio signal to the surgeon when changes in the target tissue are noted – this is an indirect assessment and less reliable than the tissue capacitance monitoring used by advanced bipolar devices to indicate endpoint has been achieved. More recently, the Harmonic ACE+7 has been released that incorporates an ‘advanced haemostasis’ mode where an algorithm controls the phases of pre-heating, vessel sealing and tissue transection. Specifically developed for larger vessel sealing and cutting, this device has been rated by the FDA to seal vessels up to 7mm in diameter.25
The tissue effects obtained with ultrasonic energy are essentially the same as those achieved with contact monopolar electrosurgery or bipolar electrosurgery, with the added function of tissue cutting. These tissue effects are achieved without the passage of electric current through the patient or the tissue grasped by the device.
Advantages of ultrasonic devices include less instrument traffic, owing to the combination of vessel-sealing and tissuecutting, and less smoke generation (a ‘mist’ of tissue debris and moisture results rather than smoke per se). The dissection capability is good, but less than that of monopolar scissors or Maryland bipolar forceps. Disadvantages include the risk of lateral thermal spread injuries, as well as higher and more prolonged instrument tip temperatures than other energy sources which could potentially result in organ injury. Also, the cutting mode, in particular, requires training and experience. As for all new-generation energy sources, ultrasonic devices are relatively expensive, but generally accessible in most institutions.
Hybrid devices
Laparoscopic devices have recently been developed that combine several energy source technologies. These include LigaSure Advance (see Figure 7; monopolar and bipolar electrosurgery; Covidien), and Thunderbeat (see Figure 8; ultrasonic and bipolar technologies; Olympus). Incorporation of multiple functionalities into a single device may reduce instrument traffic and the overall cost, although such benefits should be a secondary consideration if the individual functionalities are compromised in the hybrid configuration. Good-quality studies on the efficacy and safety of the hybrid devices are lacking.26
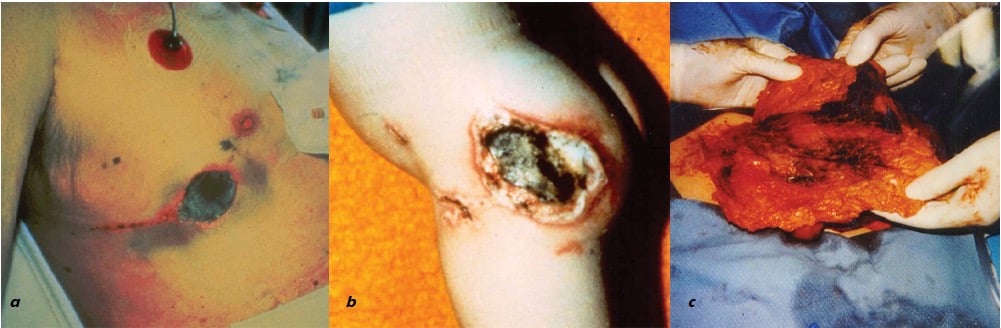
Figure 9. Types of electrosurgical injury: a) alternate path injury through an ECG electrode; b) patient return electrode burn; and c) insulation failure SCI of omentum and underlying small bowel. Types a) and b) are prevented by the mandatory use of dual-function patient return electrode pads; while entirely preventable with available technology, type c) remains a small but ever-present possibility as AEM is not mandatory.
The dangers of energy sources
Injuries owing to laparoscopic energy sources can be divided into two groups. Firstly, thereare injuries owing to surgical misadventure that occur within the surgeon’s field of vision. These include iatrogenic injuries (that is, ‘I did it’ – a mistake by the surgeon) and lateral thermal spread injuries (lateral thermal spread occurs with all energy sources). Secondly, there are stray SCIs that may be owing to capacitive coupling, insulation failure and direct coupling. These injuries occur outside the surgeon’s field of vision and are not owing to surgeon error.27
During the infancy of monopolar electrosurgery it was quickly realised that the passage of alternating current through the patient resulted in muscle contraction, cardiac arrhythmia and often death. The French biophysicist D’Arsonoval subsequently discovered, in 1891, that it is possible to pass high-frequency alternating current (<20 kHz) through the body without life-threatening ramifications.28 In 1928, the American neurosurgeon Cushing and physicist Bovie introduced their ESU that incorporated all the knowledge of the day about monopolar electrosurgery, namely, electrical energy, high-frequency alternating current with continuous and interrupted waveforms, time of application, power settings and electrode size. Their ESU remained essentially unchanged until the addition of bipolar electrosurgery technology in the 1970s.29
Although many energy sources have been introduced since the advent of laparoscopic surgery, monopolar electrosurgery remains a commonly used and cost-effective modality. However, it is unfortunately a not-infrequent cause of unexpected injury. It is estimated that injuries owing to electrosurgery occur in 0.1– 0.5 per cent of laparoscopic procedures.30 Bowel injuries owing to laparoscopy are thought to have an incidence of 1.3 per 1000 cases and, of these, half are thought to be owing to electrosurgery.31 Many, if not all, of these injuries could be prevented if surgeons understood the physics of monopolar electrosurgery and used the available technology.
Principles of monopolar electrosurgery
Current pathway
In monopolar electrosurgery, electrical current passes from the ESU to the active electrode, then through the patient to exit via a dispersive electrode, ultimately reaching ‘electrical ground’. The potential for SCI arises because electricity within the patient will take whatever pathway it can to the return to ground, including via unintended tissue targets.32 33
Fortunately, the risk of one type of SCI – the so-called alternate path injury where burns result owing to the passage of electricity through unexpected paths as it exits the patient (for example at attachment points of ECG electrodes [see Figure 9], or contact points between the patient and metal parts on the operating table) – was virtually eliminated in 1970, with the introduction of adhesive patient return electrodes connected to an isolated electrical ground within the ESU.34 35 36
Current density
The tissue effects of monopolar current relate to the current density in the tissue. Hence, focused current from the active electrode enters the patient at the site of surgery to yield a tissue effect whereas current exiting the patient via a dispersive return electrode only results in a clinically insignificant rise in tissue temperature. An injury can occur at any part of the circuit if the current density is high enough. For example, burns have previously occurred at the patient return electrode owing to poor contact with the patient’s skin, resulting high current density at the current exit point (see Figure 9). Happily, since the development of a patient return electrode that incorporates an interrogation circuit to monitor impedance increases at this site (resulting in immediate shutdown of the ESU if detected), there has not been a single verified episode of a patient return electrode burn since 1980.37 38 39
At the active electrode (or at the site of an insulation failure) a surprisingly low amount of energy is needed to cause a small bowel injury (~1mA/cm2). Consequently, the use of high power settings will also increase current density and the risk of inadvertent injury.
Waveforms
The available waveforms in monopolar electrosurgery are ‘cut’, ‘coagulation’ and ‘blend’. It is important to recognise that these terms do not imply a particular tissue effect, for example, the tissue effect is different when cut waveform is used in either contact or non-contact mode, yielding desiccation or vaporisation, respectively (see Table 2). Cut waveform is a continuous sinusoidal waveform with current flowing 100 per cent of the time, coagulation waveform is an intermittent or ‘damped’ waveform where electrical current is only delivered six per cent of the time and is off 94 per cent of the time, and blend waveforms are also intermittent waveforms, but with higher ‘on:off’ ratios. As the coagulation waveform is intermittent, a higher voltage is needed to deliver the same current to the tissue in a given time period than with cut waveform. High voltages predispose to arcing from the active electrode (or site of insulation failure) and therefore increase the risk of injury from ‘stray electric currents’. High voltages also predispose to the phenomenon of capacitive coupling.40 41
Capacitance
In simple terms, a capacitor is formed when two conductors are separated by an insulator (or dielectric). The role of the capacitor in an alternating current circuit is to conduct electrical current across the insulator. Monopolar instruments within laparoscopic ports act as capacitors: the active electrode is one conductor, the port (or biological fluid) is the other conductor and the dielectric separating the conductors is the instrument’s insulation layer. As the capacitive resistance of a capacitor is inversely proportional to the frequency of the alternating current through the circuit, the high current frequencies produced by the ESU (0.5–33 MHz) result in a low capacitive resistance.42
Mechanisms of SCI
Capacitance coupling
The high-frequency alternating current used in monopolar electrosurgery and the relatively thin instrument-insulation layer allows capacitance coupling to occur, where current from the active electrode is transmitted across the intact insulation layer (up to 62 per cent of the current delivered to the device) to another conductor nearby.43 Any nearby tissues that contact the secondary conductor are at risk of SCI. The secondary conductor could be the metal laparoscopy port – fortunately, in this scenario, the capacitive coupling current disperses safely throughout the anterior abdominal wall as it is generated.44 More concerning is the scenario with plastic laparoscopic ports: biological fluid coating the surface of the insulation layer can act as the secondary conductor and the plastic port prevents the safe dispersal of the capacitive current through the abdominal wall. In this situation, the risk of capacitive coupling injury is theoretically higher. As most hospitals have made the move to plastic laparoscopic ports, the risk of electrosurgical injury owing to capacitive coupling is an ever-present danger during laparoscopy (see Figure 10).
Insulation failure
Only a thin insulation layer surrounding the active electrode of monopolar devices protects the patient from SCI. Insulation failure occurs at sites of micro-fractures in the insulation layer, most likely owing to capacitive coupling current (and wear and tear, including the sterilisation process for reusable devices). Studies have shown between 19 and 39 per cent of re-usable hospital instruments have insulation failures.45 46 Routine sterilising department checks such as visual inspection and ‘HiPot’ testing are clearly poor predictors of insulation integrity at the end of the procedure. In addition, one of these studies showed three per cent of disposable instruments were also found to have insulation defects post-procedure.47 So, new instruments offer some, but not complete, protection against insulation failure. The most common insulation failure is a microbreakage, which is not visible to the naked eye – these small defects allow electrical current leakage of very high density, capable of producing a full-thickness visceral injury (see Figure 10).
Direct coupling
Direct coupling injury occurs when stray current from capacitive coupling or insulation failure is transmitted through another candidate to cause tissue damage. In this scenario, the laparoscope is the most likely conductor to contact other instruments and adjacent tissues.
Robot-assisted and single-port laparoscopy
The popularity of monopolar electrosurgery has been maintained with the evolution of robot-assisted and single-port laparoscopy. The risk of SCI with single-port laparoscopy and robot-assisted (particularly single- versus multi-site) laparoscopy is increased, primarily because of the surgeon’s decreased field of vision and increased risk of instrument contact/clashing. The latter increases the risk of all of capacitive coupling, insulation failure and, in particular, direct coupling SCIs.48
Diagnosis of electrosurgical injuries
SCI sustained during monopolar electrosurgery at laparoscopy is often not apparent, for example, one study reported that 69 per cent of bowel injuries were unrecognised at the time of surgery.49 The most likely explanation for this is the site of injury is usually outside the field of vision of the surgeon. These injuries typically do not present for seven to ten days after surgery. It is imperative that bowel perforation is diagnosed rapidly as it can be associated with a mortality of up to 25 per cent. Bowel perforation (and particularly small bowel perforation) may not result in overt peritonitis in the early stages, having a more indolent presentation of a low-grade temperature, moderately increased abdominal discomfort and a failure to recover at the usual rate. In this scenario a high index of suspicion of visceral injury is necessary with early investigation and early return to theatre as necessary. Delay in diagnosis substantially increases morbidity and mortality.
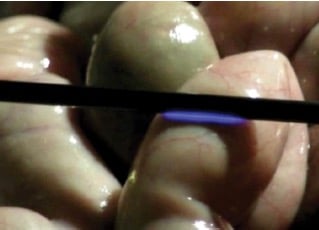
Figure 10. An animal surgery showing capacitive coupling current along the shaft of a monopolar energy source (with intact insulation) arcing to the small bowel.
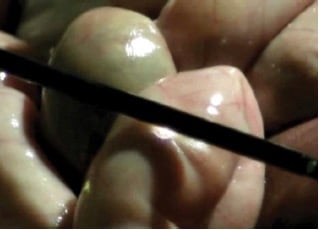
Figure 11. Insulation failure demonstrated at an animal surgery with current from the shaft of the monopolar energy source arcing to the small bowel.
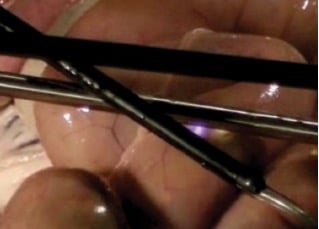
Figure 12. Direct coupling demonstrated at an animal surgery with current leaking from an insulation failure in the active electrode to the laparoscope, and then to the bowel.
Prevention of SCI
As per the above discussion, the likelihood of SCI can be reduced by: using the lowest power settings possible and shortest activation times; using ‘cut’ rather than ‘coagulation’ waveform when possible; the avoidance of tissue contact by the instrument shaft (outside the surgeon’s field of vision); the avoidance of contact with other instruments, including the laparoscope (outside the surgeon’s field of vision); and using metal laparoscopic ports. None of these measures, however, can completely eliminate the risk of SCI.50 51
Active Electrode Monitoring (AEM) was invented by Roger Odell, a former Valleylab electrical engineer, and has been commercially available since 1991 (Encision Inc). This technology performs two important tasks: capacitive coupling current is continuously channelled back to the ESU via the energy source; and an interrogation circuit triggers instant shutdown of the ESU if an insulation failure is detected.52 53 54 55 AEM is an inexpensive and available technology that uses purpose-made reusable laparoscopic instruments controlled by a proprietary box used in concert with the standard ESU (see Figure 13). AEM prevents SCI. Indeed, since the introduction of AEM, not a single SCI has been verified in surgeries that have used it. The routine use of AEM has been highly recommended by many regulatory and professional bodies.56 57 58 59 Disappointingly, the uptake of AEM in Australia and New Zealand has been low, primarily because of a lack of understanding of the technology, and a misguided belief that the technology is unnecessary. It is also important to recognise that lateral thermal spread occurs during the normal use of all laparoscopic energy sources. AEM cannot prevent lateral thermal spread injuries, just as it cannot prevent surgical errors.
Conclusion
Along with the ability to vaporise, fulgurate and desiccate tissue, as well as seal and transect vessels, the ‘ideal laparoscopic energy source’ should be capable of fine tissue grasping and sharp tissue dissection, and contain AEM technology. In addition, the combination of these functions should not come at the cost of compromising the functionality of the individual modalities.
Clearly, the ideal will not become reality any time soon!
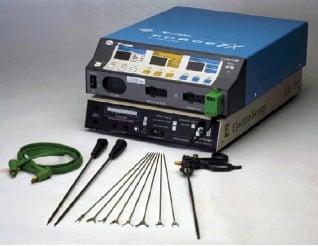
Figure 13. AEM utilises a proprietary box in concert with the ESU and reusable energy sources of which there is wide choice.
So, which energy source should the laparoscopic surgeon use? It is clear that the advent of advanced bipolar and ultrasonic vessel-sealing devices, including the hybrid energy sources, has revolutionised laparoscopy. However, despite the array of different technologies and capabilities on offer, in the battle of the vessel sealers, no clear winner has yet been decided.60 In any case, a reliance on monopolar electrosurgery persists because of its versatility; namely, the range of tissue effects and dissection capabilities. Conventional bipolar electrosurgery, too, continues to play a visible and important role.
Hence, it is likely that the surgeon will rely on two or more laparoscopic energy sources (or hybrid instruments incorporating multiple technologies) depending on the cost and availability of the devices (and their proprietary ESUs), personal preference and experience, the surgical procedure to be performed, and the presence or absence of significant pathology in the surgical field.
References
- Law KS, Lyons SD. Comparative studies of energy sources in gynaecological laparoscopy. J. Minim. Invasive Gynecol. 2013; 20: 308-31.
- Law KS, Abbott JA, Lyons SD. Energy Sources for Gynecological Laparoscopic Surgery: A Review of the Literature. Obstet. Gynecol. Survey. 2014; 69: 763-76.
- Kingston AJ, Lyons SD, Abbott JA, Vancaillie TG. Principles and practical applications of electrosurgery in laparoscopy. J. Minim. Invasive Gynecol. 2008; 15: 6. (video presentation available at www.youtube.com/watch?v=mqmvaKxfq3A).
- Law KS, Lyons SD. Comparative studies of energy sources in gynaecological laparoscopy. J. Minim. Invasive Gynecol. 2013; 20: 308-31.
- Law KS, Lyons SD. Comparative studies of energy sources in gynaecological laparoscopy. J. Minim. Invasive Gynecol. 2013; 20: 308-31.
- Law KS, Abbott JA, Lyons SD. Energy Sources for Gynecological Laparoscopic Surgery: A Review of the Literature. Obstet. Gynecol. Survey. 2014; 69: 763-76.
- Kingston AJ, Lyons SD, Abbott JA, Vancaillie TG. Principles and practical applications of electrosurgery in laparoscopy. J. Minim. Invasive Gynecol. 2008; 15: 6. (video presentation available at www.youtube.com/watch?v=mqmvaKxfq3A).
- Law KS, Lyons SD. Comparative studies of energy sources in gynaecological laparoscopy. J. Minim. Invasive Gynecol. 2013; 20: 308-31.
- Law KS, Abbott JA, Lyons SD. Energy Sources for Gynecological Laparoscopic Surgery: A Review of the Literature. Obstet. Gynecol. Survey. 2014; 69: 763-76.
- Law KS, Abbott JA, Lyons SD. Energy Sources for Gynecological Laparoscopic Surgery: A Review of the Literature. Obstet. Gynecol. Survey. 2014; 69: 763-76.
- Kingston AJ, Lyons SD, Abbott JA, Vancaillie TG. Principles and practical applications of electrosurgery in laparoscopy. J. Minim. Invasive Gynecol. 2008; 15: 6. (video presentation available at www.youtube.com/watch?v=mqmvaKxfq3A).
- Covidien Energy-based Professional Education: ‘Principles of Electrosurgery’ (www.asit.org/assets/documents/Principles_in_electrosurgery.pdf).
- Law KS, Abbott JA, Lyons SD. Energy Sources for Gynecological Laparoscopic Surgery: A Review of the Literature. Obstet. Gynecol. Survey. 2014; 69: 763-76.
- Sutton C, Abbott J. History of Power Sources in Endoscopic Surgery. J. Minim. Invasive Gynecol. 2013; 20: 271-78.
- Lyons SD, Law KS. Laparoscopic vessel sealing technologies. J. Minim. Invasive Gynecol. 2013; 20: 301-7.
- Lyons SD, Law KS. Laparoscopic vessel sealing technologies. J. Minim. Invasive Gynecol. 2013; 20: 301-7.
- Law KS, Abbott JA, Lyons SD. Energy Sources for Gynecological Laparoscopic Surgery: A Review of the Literature. Obstet. Gynecol. Survey. 2014; 69: 763-76.
- Law KS, Lyons SD. Comparative studies of energy sources in gynaecological laparoscopy. J. Minim. Invasive Gynecol. 2013; 20: 308-31.
- Lyons SD, Law KS. Laparoscopic vessel sealing technologies. J. Minim. Invasive Gynecol. 2013; 20: 301-7.
- Law KS, Abbott JA, Lyons SD. Energy Sources for Gynecological Laparoscopic Surgery: A Review of the Literature. Obstet. Gynecol. Survey. 2014; 69: 763-76.
- Lyons SD, Law KS. Laparoscopic vessel sealing technologies. J. Minim. Invasive Gynecol. 2013; 20: 301-7.
- Law KS, Abbott JA, Lyons SD. Energy Sources for Gynecological Laparoscopic Surgery: A Review of the Literature. Obstet. Gynecol. Survey. 2014; 69: 763-76.
- Lyons SD, Law KS. Laparoscopic vessel sealing technologies. J. Minim. Invasive Gynecol. 2013; 20: 301-7.
- Law KS, Lyons SD. Comparative studies of energy sources in gynaecological laparoscopy. J. Minim. Invasive Gynecol. 2013; 20: 308-31.
- Law KS, Abbott JA, Lyons SD. Energy Sources for Gynecological Laparoscopic Surgery: A Review of the Literature. Obstet. Gynecol. Survey. 2014; 69: 763-76.
- Lyons SD, Law KS. Laparoscopic vessel sealing technologies. J. Minim. Invasive Gynecol. 2013; 20: 301-7.
- Lyons SD, Law KS. Laparoscopic vessel sealing technologies. J. Minim. Invasive Gynecol. 2013; 20: 301-7.
- RC Odell. Surgical Complications Specific to Monopolar Electrosurgical Energy: Engineering Changes That Have Made Electrosurgery Safer. J. Minim. Invasive Gynecol. 2013; 20: 288-98.
- RC Odell. Surgical Complications Specific to Monopolar Electrosurgical Energy: Engineering Changes That Have Made Electrosurgery Safer. J. Minim. Invasive Gynecol. 2013; 20: 288-98.
- Wu MP, Ou CS, Chen SL, Yen E, Rowbotham R. Complications and recommended practices for electrosurgery in laparoscopy. American Journal of Surgery, 2000; 179(1):67-7.
- Bishoff JT, Allaf ME, Kirkels W, Moore RG, Kavoussi LR, Schroder F. Laparoscopic bowel injury: incidence and presentation. J Urol. 1999; 161(3): 887-90.
- Kingston AJ, Lyons SD, Abbott JA, Vancaillie TG. Principles and practical applications of electrosurgery in laparoscopy. J. Minim. Invasive Gynecol. 2008; 15: 6. (video presentation available at www.youtube.com/watch?v=mqmvaKxfq3A).
- RC Odell. Surgical Complications Specific to Monopolar Electrosurgical Energy: Engineering Changes That Have Made Electrosurgery Safer. J. Minim. Invasive Gynecol. 2013; 20: 288-98.
- Kingston AJ, Lyons SD, Abbott JA, Vancaillie TG. Principles and practical applications of electrosurgery in laparoscopy. J. Minim. Invasive Gynecol. 2008; 15: 6. (video presentation available at www.youtube.com/watch?v=mqmvaKxfq3A).
- Covidien Energy-based Professional Education: ‘Principles of Electrosurgery’ (www.asit.org/assets/documents/Principles_in_electrosurgery.pdf).
- RC Odell. Surgical Complications Specific to Monopolar Electrosurgical Energy: Engineering Changes That Have Made Electrosurgery Safer. J. Minim. Invasive Gynecol. 2013; 20: 288-98.
- Kingston AJ, Lyons SD, Abbott JA, Vancaillie TG. Principles and practical applications of electrosurgery in laparoscopy. J. Minim. Invasive Gynecol. 2008; 15: 6. (video presentation available at www.youtube.com/watch?v=mqmvaKxfq3A).
- Covidien Energy-based Professional Education: ‘Principles of Electrosurgery’ (www.asit.org/assets/documents/Principles_in_electrosurgery.pdf).
- RC Odell. Surgical Complications Specific to Monopolar Electrosurgical Energy: Engineering Changes That Have Made Electrosurgery Safer. J. Minim. Invasive Gynecol. 2013; 20: 288-98.
- Kingston AJ, Lyons SD, Abbott JA, Vancaillie TG. Principles and practical applications of electrosurgery in laparoscopy. J. Minim. Invasive Gynecol. 2008; 15: 6. (video presentation available at www.youtube.com/watch?v=mqmvaKxfq3A).
- Covidien Energy-based Professional Education: ‘Principles of Electrosurgery’ (www.asit.org/assets/documents/Principles_in_electrosurgery.pdf).
- RC Odell. Surgical Complications Specific to Monopolar Electrosurgical Energy: Engineering Changes That Have Made Electrosurgery Safer. J. Minim. Invasive Gynecol. 2013; 20: 288-98.
- Soderstrom RM. Electrosurgical injuries during laparoscopy: prevention and management. Curr Opin Obstet Gynecol. 1994 6(3): 248-50.
- Covidien Energy-based Professional Education: ‘Principles of Electrosurgery’ (www.asit.org/assets/documents/Principles_in_electrosurgery.pdf).
- Yazdani A, Krause H. Laparoscopic instrument insulation failure: The hidden hazard. J. Minim. Invasive Gynecol. 2007; 14: 228-232.
- Montero PN, Robinson TN, Weaver JS, Stiegmann GV. Insulation failure in laparoscopic instruments. Surg. Endosc. 2010; 24: 462-465.
- Montero PN, Robinson TN, Weaver JS, Stiegmann GV. Insulation failure in laparoscopic instruments. Surg. Endosc. 2010; 24: 462-465.
- Encision Inc. Single port injuries and stray current injuries. (video available at www.youtube.com/watch?v=8a74K9E0Gc8&feature=youtu.be).
- Law KS, Lyons SD. Comparative studies of energy sources in gynaecological laparoscopy. J. Minim. Invasive Gynecol. 2013; 20: 308-31.
- Kingston AJ, Lyons SD, Abbott JA, Vancaillie TG. Principles and practical applications of electrosurgery in laparoscopy. J. Minim. Invasive Gynecol. 2008; 15: 6. (video presentation available at www.youtube.com/watch?v=mqmvaKxfq3A).
- Covidien Energy-based Professional Education: ‘Principles of Electrosurgery’ (www.asit.org/assets/documents/Principles_in_electrosurgery.pdf).
- Kingston AJ, Lyons SD, Abbott JA, Vancaillie TG. Principles and practical applications of electrosurgery in laparoscopy. J. Minim. Invasive Gynecol. 2008; 15: 6. (video presentation available at www.youtube.com/watch?v=mqmvaKxfq3A).
- Covidien Energy-based Professional Education: ‘Principles of Electrosurgery’ (www.asit.org/assets/documents/Principles_in_electrosurgery.pdf).
- RC Odell. Surgical Complications Specific to Monopolar Electrosurgical Energy: Engineering Changes That Have Made Electrosurgery Safer. J. Minim. Invasive Gynecol. 2013; 20: 288-98.
- Vancaillie TG. Active electrode monitoring. How to prevent unintentional thermal injury associated with monopolar electrosurgery at laparoscopy. Surg. Endosc. 1998; 12: 1009-1012.
- Kingston AJ, Lyons SD, Abbott JA, Vancaillie TG. Principles and practical applications of electrosurgery in laparoscopy. J. Minim. Invasive Gynecol. 2008; 15: 6. (video presentation available at www.youtube.com/watch?v=mqmvaKxfq3A).
- Tucker RD, Voyles CR. Laparoscopic electrosurgical complications and their prevention. AORN J 1995; 62(1): 51-3.
- Health Devices – ECRI. Special topics in electrosurgical safety: managing burn risks during laparoscopic and high-current procedures. Health Devices 2005; 3498: 257-72.
- American Association of Gynecological laparoscopy. (1995) A technical bulletin: Electrosurgical safety (AAGL) 1995.
- Lyons SD, Law KS. Laparoscopic vessel sealing technologies. J. Minim. Invasive Gynecol. 2013; 20: 301-7.
Leave a Reply